ABSTRACT
Silicon carbide (SiC) is a versatile industrial material and has been in use in various spheres because of its distinctive electrical and thermal properties. Among various fields, it has contributed significantly to biosensor technologies because it is both bio and hemo-compatible. Of particular interest in this study is its potential for biomedical applications. Although about 907 SiC-based biosensor articles have been published in the past 20 years (2000–2022), not many review articles have encapsulated the advancement and uses of the SiC-based biosensor. In order to better understand the development stage, research hives, and future advancement trends of SiC-based biosensor technology, it is essential to perform a broad retrospective investigation. The present study aims to explore the potential of SiC-based biosensors and their distinct features for interdisciplinary collaborations, utilizing machine learning through big data analytics. By reviewing research in this field, the study seeks to uncover global trends, challenges, and gaps in SiC-based biosensor research, as well as identify new research areas and technologies that could advance biosensor technology. By conducting the analysis, this study provides insights into the most impactful articles and top contributors to biosensor research, including journals, authors, institutions, and countries. Additionally, the study examines the methodological approaches and research contexts employed in this field. Results suggest that one of the evolving technological paths in biosensors is the use of silicon carbide-based biosensors and medical devices, which in turn are poised to transform into the commercial sphere. The main characteristics of these emerging research grounds and technologies in biosensors are illustrated for the productive effects of research and innovation policy that led to scientific advances and technological change in society.
INTRODUCTION
Biosensors play a crucial role in gauging biological or chemical reactions by generating signals proportional to the strength of an analyte. While the exact origins of biosensors are not definitive, significant advancements were made over half a century ago. Clark and Lyons introduced the first primitive biosensor, which monitored blood gas contents during surgical procedures.[1] Since then, continuous efforts have been made to develop new biosensors for diverse applications, such as heart stents, dental implants, home pregnancy tests, glucose detectors, environmental monitoring etc.[2–10] Thanks to dedicated scientific efforts, biosensors have undergone remarkable advancements as a technology leading to a significant expansion in their capabilities, increased sensitivity, and a broader range of applications.[1–17]
Further advancement resulting in novel sensing techniques have significantly improved the performance of biosensors. One significant aspect of progress lies in the improvement of biosensor selectivity. The integration of nanomaterials, including nanoparticles, nanowires, and nanotubes, has enabled the creation of highly sensitive biosensors capable of detecting analytes at extremely low concentrations.[18] Additionally, progression in molecular biology and genetic engineering have facilitated the incorporation of biological components, such as enzymes and antibodies, into biosensors, enabling the detection of specific targets.[19]
Silicon planar technology has driven the miniaturization and integration of biosensors. By leveraging advancements in microfabrication techniques, researchers have successfully developed portable and cost-effective miniaturized biosensors that can be seamlessly integrated into various devices and systems.[20] This integration has expanded the accessibility and applications of biosensors manifold, enabling real-time monitoring and personalized healthcare.
Furthermore, scientific research has explored and utilized novel detection principles, expanding the capabilities of biosensors beyond traditional analytical methods. For instance, research in the field of plasmonics has led to the development of Surface Plasmon Resonance (SPR) biosensors, which utilize the interaction between light and metallic nanostructures to detect and analyze biomolecular interactions in real-time, offering highly sensitive detection capabilities.[21] Similarly, research on Electrochemical Impedance Spectroscopy (EIS) has resulted in the creation of label-free biosensors that detect biomolecular interactions based on changes in electrical impedance.[22]
Advancements in signal transduction have also been driven by scientific research, enabling more accurate and rapid detection in biosensors. The integration of optical technologies, such as fluorescence, luminescence, and Raman spectroscopy, has provided highly sensitive and specific detection capabilities in biosensors.[22] Moreover, improvements in electronics and signal processing techniques have contributed to the development of biosensors with enhanced signal amplification, noise reduction, and data analysis capabilities.[23] These biosensors, when integrated with compatible materials, offer a sophisticated platform capable of long-term solutions for detecting and analysing multiple biomolecules on a single chip within the challenging environment of the human body.[24–26]
In the field of biomedical applications, SiC-based biosensors have garnered significant interest, driven by scientific research. Silicon carbide, known for its durability and hardness, has emerged as the most widely used active biomaterial in the 21st century.[25–30] The growing number of international collaborations, emerging publications, and research activities dedicated to SiC-based biosensors reflects this trend.[25–30] While numerous review articles have summarized achievements, explored techniques, and discussed future challenges and prospects,[24,30-41] this study aims to provide a distinct perspective. By employing bibliometric analysis, the authors seek to illustrate the historical landscape and utilization of SiC in biosensors, offering insights beyond the technical content presented in existing reviews.
This research article aims to provide a comprehensive review of SiC-based biosensors using bibliometric analysis, focusing on their unique properties, potential for interdisciplinary collaborations, and contributions to the evolution of biosensor technology. By identifying emerging research areas and technologies, the article aims to uncover global trends, gaps, and challenges in SiC-based biosensor research. It highlights the advantages of SiC-based biosensors and acknowledges the significant contributions of research articles, journals, authors, institutions, and countries in this field. Additionally, the article encourages researchers to explore in vivo applications of SiC-based biosensors and offers valuable insights into important publications and emerging sub-branches. Ultimately, the goal is to accelerate research, boost scientific productivity, foster collaborations, and promote innovation and multidisciplinarity.
To achieve these objectives, the study employs bibliometric technologies to quantitatively and qualitatively examine the research developments in SiC-based biosensors. The Scopus database, widely recognized for its comprehensive coverage of reputable scientific journals and publications, is utilized as a strong foundation for exploring and understanding advancements, trends, and contributions in the field of biosensors.
The study addresses specific research questions that are strongly grounded in the research objectives. These include investigating the changing trends in SiC-based biosensor publication over time, identifying the most active authors, countries, and journals in the field, examining highly cited articles, determining core keywords in biosensor publications, and exploring identifiable sub-domains within SiC-based biosensor research.
The paper is structured as follows: Section 2 provides detailed information on the data source, search strategy, and analysis methods employed. Section 3 presents a comprehensive discussion of the findings, while Section 4 concludes the paper and highlights prospects for further expansion and research.
METHODOLOGY
This study gathers bibliometric data on SiC based biosensor research for its review. To do so, this study follows and employs the Scientific Procedures and Rationales for Systematic Literature Reviews (SPAR-4-SLR) protocol, which consists of three major stages, namely assembling, arranging, and assessing of articles.[42]
Assembling
To assemble the number of articles on Silicon carbide-based biosensors, the authors identified the search keywords related to SiC based biosensors from a primary review of relevant literature and consulted 10 experts to establish the appropriateness of those keywords to represent SiC based biosensors. This led to a combination of 9 keywords that are listed in the following search string:
{Silicon carbide} AND (“Biosensor” OR “Biosensing” OR “biomaterial” OR “microfluidics” OR “biomimetic” OR “nanomaterials” OR “nanofabrication” OR “bioelectronics” OR “biochip”).
Using the aforementioned search keyword string, relevant articles on the Scopus database are searched, as Scopus is the largest high-quality scientific database of scholarly articles,[43,44] and thus preferred over its alternative, Web of Science, which contains fewer articles for review.[42] It may be noted that, while adhering to the SPAR-4-SLR protocol for assembling data, the authors took care to include significant and relevant literature for the study, and all the literature used is referenced in the appropriate section of the manuscript. In all, 907 documents were returned from the search.
Arranging
The 907 articles retrieved from the search results are then arranged and filtered using the category (code) function in Scopus according to the publication year – “till 2021”, document type-”article”, publication stage-”final”, source type-”journal” and language-”English” type respectively. These filters were applied coherent with the recommendations made by Paul[42] because non-articles such as editorials and notes may not be refereed and the presence of reviews can result in dual barred comprehension. In-press articles were not considered as they were not confirmed; non-journal sources such as books, book chapters, and conference proceedings were left out as they may not have gone through a rigorous peer review process; articles published in languages other than English were excluded because of limited language proficiency in these languages. All this has reduced the number of articles to 550. These 550 articles are then downloaded and read, removing another 6 articles that mention the keywords sparingly.
Assessing
To assess the final corpus of 550 articles on SiC-based biosensors, which is relatively a large corpus, this study adopts a bibliometric analysis approach for its review. The bibliometric analysis comprises performance analysis, science mapping, and network analysis. The performance analysis provides insight on the publication trend, the top articles, and contributing journals, authors, and institutions. Temporal analysis using word clouds delineates the science mapping and to take out the major themes and topics behind the intellectual structure of SiC-based biosensor research,[45] network analysis is done using keyword co-occurrence in VOSviewer.[45–50] This study curates a future research outline based on our reading of the articles and reflection of existing gaps under each major theme in order to broaden understandings in the field. The next section presents the results of the review, wherein descriptions are accompanied by figures and tables.
FINDINGS
Performance Analysis
Performance analysis is a bibliometric investigation method that explains the functioning of a research area,[48] and here, the field of SiC-based biosensors. This investigation is parallel to that of the profiling of participants in observed studies, even though in a more arduous way via the use of bibliometric metrics.[48] In the present study, a performance analysis is steered to disclose (1) the publication trend, (2) the most influential articles, the top contributing (3) journals, (4) authors, (5) institutions, and (6) countries in the context of SiC-based biosensors.
Publication trend for SiC based biosensors research
The first publication on SiC-based biosensors in a Scopus indexed journal emerged in 1991,[51] and since then, there has been a consistent increase in publications over the course of 31 years (1991-2021). Figure 1 illustrates the analysis of the publication trend using a three-year moving average. Initially, from 1991 to 2001, the number of publications remained relatively low, with only one or two articles published annually. This period can be characterized as a phase of steady but gradual growth. It can be seen that around 2002, a significant shift occurred in the trend. The three-year moving average began to display an upward slope, indicating an accelerated growth in SiC-based biosensors research. Over the next 15 years, there was a substantial increase in publications, reaching a peak of 34 average publications in 2015. This period marks a phase of rapid expansion within the field. Another noteworthy change in the publication trend took place around 2019. The three-year moving average exhibited a steeper incline, suggesting the onset of an exponential growth phase. This shift can be attributed to improvement in engineering techniques, enabling the fabrication of robust SiC materials.[52–54] The availability of such materials in laboratory settings facilitated their exploration for biomedical applications, leading to a surge in research activity and breakthroughs in SiC-based biosensors. Between 2009 and 2015, the growth remained relatively steady, following a linear pattern.
However, from 2015 to 2018, the three-year moving average experienced a decline of approximately 10 publications per year from its peak value. This decline could be attributed to researchers dedicating more time to in-depth investigations and exploration of various fabrication techniques for SiC materials in biosensing applications. After 2019, there was a notable resurgence in the publication trend, characterized by a sharper upward trajectory.

Figure 1:
Publication trend for Silicon carbide based biosensors 1991-2021
This indicates a more rapid expansion of SiC-based biosensors research. This phase of exponential growth might be attributed to increased awareness, funding, and collaborations within the field.
In summary, the publication trend of SiC-based biosensors research shows a gradual growth phase initially, followed by an accelerated growth period and subsequent exponential expansion. The trend has been influenced by scientific research and advances fabrication technology, development in engineering techniques, and the availability of funding and collaborations. Given the notable growth and increasing research activity in SiC-based biosensors over the years, it is reasonable to anticipate continued progress and exploration in this field which will result in better gadgets and its application in varied fields.
Most influential articles for SiC based biosensors research
Table 1 shows the ten most influential articles for SiC-based biosensors research in terms of citations. According to the table, Fang, Bando, Gautam, Ye, and Golberg’s (2008) article is the most cited in the field, with an average of 39 citations per year and a total of 590 citations since its publication in 2008.
This is followed by the articles “A nanocomposite ultraviolet photodetector based on interfacial trap-controlled charge injection” by Guo, Yang, Yuan, Xiao, Dong Bi and Huang (2012) and “Microwave-assisted chemistry: Synthetic applications for rapid assembly of nanomaterials and organics” by Gawande, Shelke, Zboril and Varma (2014), which have been cited 536 and 414 times, respectively. Interestingly, the top three most-cited articles in the field are about nanostructures and nanomaterials, demonstrating their importance in the field. Notably, the top 10 most-cited articles in the field have received a total of 3054 citations, demonstrating the significant impact of SiC-based biosensors research in the scientific community.
Top contributing journal for SiC based biosensors research
The corpus of 550 articles on SiC-based biosensors was published across 291 journals, with Table 2 showing that the top 10 contributing journals with at least six articles on silicon carbide published 82 (15%) articles in the field. The Journal of Crystal Growth, Materials Letters, and Ceramics International are the top three most prolific journals, with 18, 15, and 13 articles, respectively. However, in terms of influence, Nano Letters leads the pack with 359 citations, followed by ACS Nano and Chemical Communications, each with 291 and 281 citations. Most of the articles in these publications have focused on the characterization and analysis of SiC-nanostructures suitable for sensor applications,[55,56] the design and optimization of biosensor devices using SiC[57] and the functionalization of SiC surfaces to enhance biosensing capabilities.[58]
Author(s) | Article title | Year | TotalCitations (TC) | TC per Year |
---|---|---|---|---|
Fang X., Bando Y., Gautam U.K., Ye C., Golberg D. | Inorganic semiconductor nanostructures and their field-emission applications. | 2008 | 590 | 39 |
Guo F,, Yang B., Yuan Y., Xiao Z., Dong Q., Bi Y., Huang J. | A nanocomposite ultraviolet photodetector based on interfacial trap-controlled charge injection. | 2012 | 536 | 49 |
Gawande M.B., Shelke S.N., Zboril R., Varma R.S. | Microwave-assisted chemistry: Synthetic applications for rapid assembly of nanomaterials and organics. | 2014 | 414 | 46 |
Sprinkle M., Ruan M., Hu Y., Hankinson J., Rubio-Roy M., Zhang B., Wu X., Berger C., De Heer W.A. | Scalable templated growth of graphene nanoribbons on SiC. | 2010 | 375 | 29 |
Zhang Z., Han X.D., Zhang Y.F., Zheng K., Zhang X.N., Hao Y.J., Guo X.Y., Yuan J., Wang Z.L. | Low-temperature in situ large strain plasticity of ceramic SiC nanowires and its atomic-scale mechanism. | 2007 | 229 | 14 |
Aboushelib M.N., De Jager N., Kleverlaan C.J., Feilzer A.J. | Microtensile bond strength of different components of core veneered all-ceramic restorations. | 2005 | 223 | 12 |
Lan F., Demaree B., Ahmed N., Abate A.R. | Single-cell genome sequencing at ultra-high- throughput with microfluidic droplet barcoding. | 2017 | 204 | 34 |
Su L., Wang H., Niu M., Fan X., Ma M., Shi Z., Guo S.-W | Ultralight, Recoverable, and High-Temperature- Resistant SiC Nanowire Aerogel. | 2018 | 171 | 34 |
Lee T.-H., Bhunia S., Mehregany M. | Electromechanical computing at 500°C with silicon carbide. | 2010 | 166 | 13 |
Li Y., Li F., Zhou Z., Chen Z. | SiC2 silagraphene and its one-dimensional derivatives: Where planar tetracoordinate silicon happens. | 2011 | 146 | 12 |
Journal | TP | TC |
---|---|---|
Journal of Crystal Growth | 18 | 247 |
Materials Letters | 15 | 275 |
Ceramics International | 13 | 113 |
Acs Applied Materials and Interfaces | 9 | 264 |
Journal of Alloys and Compounds | 9 | 157 |
Chemical Communications | 8 | 281 |
Journal of Physical Chemistry C | 8 | 206 |
Biosensors and Bioelectronics | 7 | 251 |
Journal of Biomedical Materials Research – Part B Applied Biomaterials | 7 | 239 |
Nature Communications | 6 | 253 |
Author | TP | TC | TC/TP |
---|---|---|---|
Li Y | 13 | 319 | 24.5 |
Wang L | 13 | 296 | 22.7 |
Zhang X | 12 | 358 | 29.8 |
Li X | 11 | 274 | 24.9 |
Li H | 10 | 124 | 12.4 |
Liu X | 10 | 101 | 10.1 |
Wang Y | 10 | 141 | 14.1 |
Yang Y | 10 | 327 | 32.7 |
Chen J | 9 | 388 | 43.1 |
Wang H | 9 | 298 | 33.1 |
Top contributing authors for SiC based biosensors research
Table 3 lists the top ten contributing authors for SiC-based biosensor research. According to the table, Li Y. from the University of Birmingham in the United Kingdom and Wang L. from Ningbo University of Technology in China are the two most prolific authors in the field, each with 13 articles. Zhang X. from Ningbo University of Technology, China, and Li X. from Harbin Institute of Technology, China, came in second and third, with 12 and 11 articles, respectively.
The most influential authors, however, are Chen J. from the China Academy of Launch Vehicle Technology, China, and Zhang X. from Ningbo University of Technology, China, with 388 and 358 citations, respectively, though the latter (TC/TP = 43.1) has a higher average return of citations per year than the former (TC/TP = 29.8). Taken collectively, the top 10 contributing authors for SiC-based biosensors research have contributed a total of 94 (17.1%) articles that have amassed 2307 citations in the field.
Top contributing Countries for SiC based biosensor research
The top ten contributing countries for SiC-based biosensors research are presented in Table 4. According to the table, China is the most prolific country, with 172 articles, followed by the United States and Germany, with 86 and 26 articles, respectively. China and the United States also emerge as the top two most influential countries, with 3,536 and 3,240 citations, respectively, and are joined by Germany, which ranks third with 1,247 citations. While Asian, American and European countries dominate the list of the top 25 contributing countries, there is notable representation from Oceanic countries such as Australia. Despite this representation, only 22 out of 550 articles used samples from Australia, indicating that the majority of research on SiC-based biosensors is still focused on Asia, America, and Europe. Nonetheless, detailed examination reveals that SiC-based biosensors research in Australian countries began to emerge more prominently in the decade 2006–2016, a trend that should and is likely to continue in the future.
The highly cited articles and the top contributing authors from various countries for SiC based biosensors reveals ongoing research efforts in the fabrication of nanowires or nanostructure-based SiC biosensors for diverse biomedical applications.[14–16] Additionally, researchers are leveraging the inherent hardness and robust nature of SiC to develop various bio supports.[59–62]
Country | TP | TC | TC/TP |
---|---|---|---|
China | 172 | 3536 | 20.6 |
USA | 86 | 3240 | 37.7 |
Germany | 26 | 1247 | 47.9 |
Japan | 25 | 852 | 34.1 |
Australia | 22 | 554 | 25.2 |
France | 19 | 454 | 23.9 |
India | 17 | 430 | 25.3 |
Italy | 17 | 424 | 24.9 |
Korea | 16 | 376 | 23.5 |
Spain | 13 | 304 | 23.4 |
Science Mapping
Science mapping is an analysis that discloses and provides a graphical representation of what knowledge exists and how it is interconnected in a domain, in this case, SiC-based biosensors.[48] The science mapping of SiC-based biosensors research is carried out in VOSviewer using two bibliometric analysis techniques: a temporal analysis using word clouds to unpack the major topics characterising SiC-based biosensors research across each time period; and a network analysis using keyword co-occurrence to reveal the major themes underpinning the intellectual structure of SiC-based biosensors research over the last 35 years (1987–2022).
Temporal analysis using word clouds for SiC based biosensor research
The corpus of SiC-based biosensors research articles was divided into four time periods: 1987 to 1994; 1994 to 2001; 2001 to 2015; and 2015 to 2022. The word clouds in Figures 2, 3, 4, and 5 depict the major topics revealed through temporal analysis in each time period.
Silicon carbide research began with its synthesis and processing to understand the various properties that it can be used for.[63] Figure 2 depicts the property of silicon carbide as a hemo-compatible material. As a result, between 1987 and 1994, the first years of research focused on silicon carbide and its potential in biological applications. Because of its blood compatibility, it was used in studies involving blood and bones, bringing to light keywords such as “blutgerinnung”-blood clotting, “fibrinogen”, “fibroblasts”, “clotting proteins”, “blutverträglichkeit”-blood compatibility, and “osteoblasts”.[64]
Figure 3 illustrates the continued growth of SiC-based biosensors from 1994 to 2001 through the exploration of new areas such as its “biocompatible” nature and “low fusing” capability, indicating SiC’s chemical inertness. Because of its high hardness,
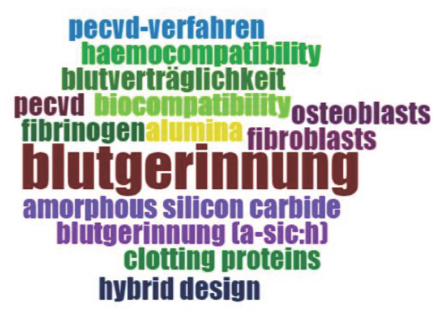
Figure 2:
Silicon carbide based biosensors research between 1987 and 1994
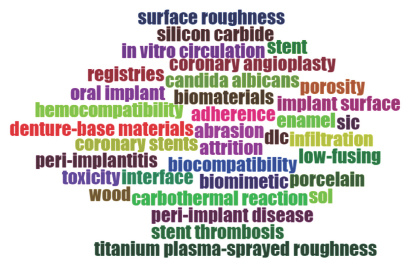
Figure 3:
Silicon carbide based biosensors research between 1994 and 2001
“adherence,” and lower “toxicity,” it was used in “stenting” therapy (Amon et al., 1996). For example, the use of silicon-carbide coated “coronary” “stents” caused low platelet and leukocyte “adhesion” during platelet activation.[65] It also aided in the reduction of “thrombosis” during stenting treatments.[66] As a result, the robust nature began to be heavily utilised during these years, providing an initial boost in the advancement of SiC-based biosensor research.
Figure 4 demonstrates the accelerating growth of SiC in the domain of nanotechnology between 2001 and 2015. SiC began to be widely used in “nanotubes,” “nanofabrication,” “nanowires,” “nanostructures,” and other applications.[67] Various other engineering applications of silicon carbide “nanoparticles” and microparticles were investigated during this time period. [68] In order to maximise the use of SiC, several techniques and phenomena, including “photoluminescence,” “chemical vapour deposition,” “crystallography,” and many others, were studied concurrently. As a result, there has been a significant increase in the research of SiC-based biosensors during this time period, as also illustrated in Figure 1.
Finally, Figure 5 indicates the continued growth of silicon carbide in nanomaterials and nanotechnology. The use of “graphene” in conjunction with “silicon carbide” for the production of “biosensors”[38] and other applications has given continuous rise in the field, owing to the improvement of the “mechanical properties” of the obtained structures and better performance in their “thermal”, “chemical,” and physical properties. It also resulted in the expansion of applications for SiC-based biosensors in biomedical devices.[69] As a result, the time period 2015–2022 has given a boost to the growth of SiC-based biosensor research, which trend is expected to continue in the future.
Network analysis
Figure 6 shows a co-occurrence network map generated from the publications of the authors. This research work presents and visualises a co-authorship bibliometric network using the terms
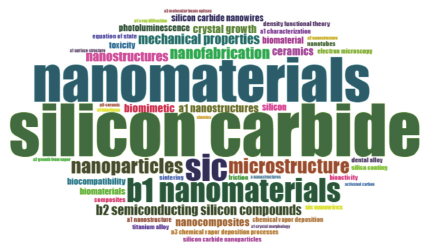
Figure 4:
Silicon carbide based biosensors research between 2001 and 2015

Figure 5:
Silicon carbide based biosensors research between 2015 and 2022

Figure 6:
Co-occurrence network map generated from publications of the authors
“citation” and “author.” Accordingly, the minimum number of research papers and the citations received for an author were fixed at 5 and 39, respectively, in the VOSviewer. Finally, 36 authors out of 2496 met the minimum thresholds. A map contains several components, including author nodes (circles), co-occurrence weight (circle size), networked relationship clustering (colour and proximity), and author names (text). The size of the node and the density of “links” to other authors indicate the author’s importance in the literature. Citations shared by these authors are represented by links between them. Co-authorship relationships
indicate whether an author wrote a paper in collaboration with another author in the field. It investigates the interactions of researchers in the field. Because co-authorship is a formal way for scholars to collaborate intellectually,[70,71] it is critical to understand how scholars interact with one another. Analysing co-authorship data will aid in identifying groups of people who can collaborate closely and further contribute to the field of bio-sensors more effectively. The analysis conducted in this study identifies Zhang X., Chen J., and Wang L. as highly influential authors in the field of SiC-based biosensors. These authors have made substantial contributions to the field. The theories and concepts proposed by them should be taken into consideration by researchers, industries working in this area as well as by beginners planning to enter into the field of bio-sensors. Collaborating with influential authors like Zhang X., Chen J., and Wang L. can provide valuable benefits for researchers seeking to utilize their expertise in various aspects of SiC-based biosensors, including material characterization, surface functionalization, signal transduction mechanisms, novel sensor designs, fabrication techniques, and innovative applications.[15,16] Through collaboration with these experts, researchers can leverage their knowledge and experience to expedite their own research progress and contribute to the advancement of SiC biosensors.
Additionally, the collaborations between Chinese authors and researchers from other countries have played a significant role in the notable growth observed in the field of SiC-based biosensors worldwide.[15-17,19,60] This highlights the importance and impact of international collaborations in advancing research and promoting global advancements in SiC biosensors.
Figure 7 depicts the VOS Viewer scientometric analysis, which included countries with a minimum of 5 articles and 50 citations. A total of 32 countries out of 64 met the threshold. In this view, countries are indicated by a label and by a circle. The more significant a country, the larger its label and circle. Each circle’s size represents the number of papers written by authors from that country. Each link connecting two circles from different countries indicates that the organisations in those countries worked together on the project. The collaboration patterns identified in Figure 7 highlight the relationships between countries based on co-authorship networks. Countries like China, the United States, and Germany have established strong co-authorship relationships, indicating active collaborations and knowledge exchange.
Chinese authors collaborated with authors from other countries, but not as much as authors from the United States, indicating that many Chinese authors collaborated within the country. It is clear that Asian, North American, and European countries contribute the most out of the seven continents. China leads the Asian countries with 186 articles published some in collaboration with international researchers. The United States has produced approximately 238 articles among North American countries, mostly in collaboration with a total of 23 other countries. Finally,

Figure 7:
VOS Viewer scientometric analysis illustrating the network between countries
Germany leads the European Union in the production of articles based on SiC-based biosensors.
Countries such as Belgium, Malaysia, Turkey, and Romania have the fewest collaborations with other countries and thus have contributed the least in the research field, with an average of 5 documents each.
In the field of SiC-based biosensors, both the United States and China are actively engaged in research and development, focusing on various aspects of this technology. In the United States, researchers are involved in the development of advanced SiC materials with improved properties for biosensing applications. They explored novel fabrication techniques and surface functionalization methods to enhance the performance of SiC-based biosensors.[24,26,39,72-74] Additionally, studies concentrated on sensor design and fabrication, aiming to optimize the structure and electrode configurations to achieve enhanced sensitivity, selectivity, and stability.[24,26,39,72-74] Similarly, in China, SiC-based biosensor research is thriving. Researchers focussed on material synthesis and modification, working on tailoring the properties of SiC materials and exploring surface modification techniques to enhance biosensor performance.[15-16,75] Sensor development and optimization are also key areas of research, with efforts directed towards improving fabrication techniques, sensor integration, and miniaturization for portable or implantable applications.[76,77] Biofunctionalization strategies are investigated to immobilize biomolecules effectively on SiC surfaces, enabling enhanced biorecognition and analyte capture.[76,77] Chinese researchers are also keen on developing SiC biosensors for point-of-care applications, with an emphasis on creating portable and cost-effective devices. This involves exploring novel sensing mechanisms, miniaturization strategies, and integration with microfluidic systems.[77]
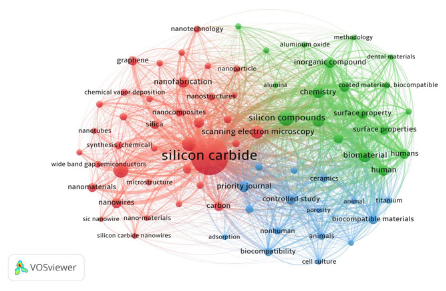
Figure 8:
Keyword network map with minimum no. of occurrences = 20
Ongoing studies in the United States, China, and other countries contributed in advancing the understanding and applications of SiC-based biosensors. The research efforts in both the United States and China underscore the significance of SiC-based biosensors in various domains and their potential impact on biomedical applications. As a result, the authors of other countries must collaborate with China, the United States, Germany, France, and others if they plan to expand their growth in the field of SiC-based biosensors. These collaborations will definitely result in more advancement of SiC-based biosensors research by fostering sharing of ideas, expertise, and resources.
In Figure 8, an aggregation of words in the three predominant colours of red, green, and blue is shown. The terms presented in the figure in red, such as silicon carbide, nanostructured materials, nanoparticles, nanotechnology, silicon carbide nanowires, and nanofabrication, represent elements that make up the layers of data collection and aggregation. The terms “silicon carbide” and “nanostructured materials” in red and “biomaterial” in green, which are linked to many other terms in the graph, bring an understanding that the main theme of the research articles addresses silicon carbide, bringing a direct relationship with biomaterials and nanostructures.
DISCUSSION
SiC-based biosensors have gained significant attention for their exclusive properties and potential biomedical applications. Scholars from both developed and developing countries have actively explored and optimized the properties and structure of silicon carbide for biosensing purposes.[1–30] The increasing publication trend in this area indicates a growing interest and the potential for further advancements (Figure 1). Future researchers can benefit from the expanding body of knowledge, advancements in engineering techniques, diverse research focuses, and increased support through funding and collaborations. This favourable position will enable them to delve deeper into SiC materials, uncover new functionalities, and develop innovative biosensing approaches. Moreover, future researchers may benefit from improved fabrication methods, enhanced sensitivity, selectivity, stability, and a wider range of biomedical applications for SiC-based biosensors.
However, despite the progress made, there are several gaps in the existing research that warrant further investigation. Scalability and cost-effectiveness are crucial considerations for the widespread adoption of SiC biosensors. Exploring cost-effective manufacturing techniques, scalable fabrication processes, and methods for mass production will make SiC biosensors more accessible and affordable for biomedical applications.[26,27] Additionally, future research should focus on expanding the capabilities of SiC biosensors to enable real-time monitoring and multi-analyte detection. Advancements in real-time monitoring and the ability to detect multiple analytes simultaneously would facilitate comprehensive and dynamic monitoring of biological processes and disease conditions. Lastly, the application of SiC biosensors in in vivo settings requires additional exploration. While most research has been conducting in vitro or ex vivo, understanding the challenges associated with implantable SiC-based biosensors and their interaction with living tissues is crucial for their successful implementation in biomedical diagnostics and therapeutics.[26,27]
Addressing these gaps through focused research efforts and by establishing more international collaborations will contribute to the advancement of SiC-based biosensors and expand their potential applications in the field of biomedicine.
CONCLUSION
This study utilizes a systematic literature review approach, incorporating bibliometric analysis, to provide valuable insights into the performance and scientific landscape of SiC-based biosensors. The methodology employed in this research exemplifies the application of big data analytics, to identify influential articles, top contributing entities (journals, authors, institutions, and countries), and the evolving research themes and contexts related to silicon carbide-based biosensors. By examining 550 articles published between 1991 and 2021, the study highlights four key findings and their implications.
Firstly, the analysis reveals a consistent growth in publications since 2002, primarily driven by advancements in SiC substrate fabrication. Notably, around 70% of the articles were published between 2009 and 2020, with the first publication on SiC biosensors dating back to 1991. The most cited article, titled “Inorganic semiconductor nanostructures and their field-emission applications” by Fang X. et al. in 2008, received 590 citations. The study also presents a list of the top 10 most cited publications in the field, which collectively garnered 3054 citations. Furthermore, it identifies the top 10 journals publishing SiC-based biosensor research, with these journals contributing 82 articles, representing 15% of the total publications.
Secondly, the analysis indicates that most studies focus on the fabrication of SiC substrates, films, or nanostructures, rather than application-oriented research. Journal statistics reveal that the Journal of Crystal Growth has published the highest number of articles (18) in this area, with significant impact as evidenced by 247 total citations. Notably, recent journals such as Nano Letters, ACS Nano, and the Journal of the American Ceramic Society have also made notable contributions, despite their relatively fewer publications (5, 4, and 5, respectively), with citation counts of 359, 291, and 233 respectively. The top 10 authors collectively contributed 17.1% of the total publications. Geographically, research in this field is predominantly concentrated in China, the USA, and European countries, with India ranking as the seventh leading nation. The study suggests that future researchers should seize these gaps as opportunities to explore in vivo applications of SiC-based biosensors.
Thirdly, the temporal analysis of science mapping reveals the evolution of SiC-based biosensors research, starting with a focus on its hardness and robust nature, and progressively branching out to encompass areas such as nanostructures, biocompatibility, and hemocompatibility. The study suggests that the field of SiC-based biosensors will continue to expand in the future, with the development of advanced biomedical instruments. This information indicates potential research gaps (like scalability and cost effectiveness, more in vivo studies, detection of multianalyte, etc.) or emerging areas that require further exploration, enabling researchers to address these gaps and contribute to the evolving research landscape.
Lastly, the network analysis of keyword co-occurrences highlights the strong connection between silicon carbide-based biosensors and nanostructures. This bibliometric investigation, conducted over the past 20 years, provides valuable insights into significant publications, leading authors, and emerging sub-branches in the field. The study serves as a useful resource for established research groups, beginners and individuals with interests in SiC biosensors, accelerating research progress and fostering collaborations. Furthermore, it paves the way for innovation and multidisciplinary approaches by facilitating interactions among authors and institutions, leading to the emergence of new ideas.
Overall, this comprehensive review of SiC-based biosensors using bibliometric analysis contributes to the existing knowledge by offering a distinct perspective. By employing quantitative and qualitative analysis methods, the study goes beyond the technical content of existing reviews, uncovering global trends, identifying gaps and challenges, and recognizing the contributions of articles, journals, authors, institutions, and countries. The study emphasizes the advantages of SiC-based biosensors and encourages researchers to explore their potential applications in in vivo settings. By providing a comprehensive overview, the study aims to accelerate research progress, enhance scientific productivity, foster collaborations, and promote innovation and multidisciplinary.
Cite this article
Talwar J, Bhardwaj A, Soni ND. Global Trends in Silicon Carbide Biosensor Research: A Bibliometric Study. J Scientometric Res. 2023;12(2):372-82.
References
- Clark LC, Lyons C. Electrode Systems for Continuous Monitoring Cardiovascular Surgery. Annals of the New York Academy of Sciences. 1962;102:29-45. [CrossRef] | [Google Scholar]
- Al-madani H, Du H, Yao J, Peng H, Yao C, Jiang B, et al. Living Sample Viability Measurement Methods from Traditional Assays to Nano motion. Biosensors. 2022;12(7):453 [CrossRef] | [Google Scholar]
- Baeumner AJ. Biosensors for environmental pollutants and food contaminants. Analytical and Bioanalytical Chemistry. 2003;377:434-45. [CrossRef] | [Google Scholar]
- Bhalla N, Jolly P, Formisano N, Estrela P. Introduction to biosensors. Essays in Biochemistry. 2016;60(1):1-8. [CrossRef] | [Google Scholar]
- . Over-the-Counter Biosensors:Past, Present, Future. Sensors. 2008;8(9):5535-59. [CrossRef] | [Google Scholar]
- Li YJ, Lu CC. A Novel Scheme and Evaluations on a Long-Term and Continuous Biosensor Platform Integrated with a Dental Implant Fixture and Its Prosthetic Abutment. Sensors. 2015;15(10):24961-76. [CrossRef] | [Google Scholar]
- Rodriguez-Mozaz S, Alda LMJ, Barcelo D. Biosensors as useful tools for environmental analysis and monitoring. Analytical and Bioanalytical Chemistry. 2006;386:1025-41. [CrossRef] | [Google Scholar]
- Terry LA, White SF, Tigwell LJ. The Application of Biosensors to Fresh Produce and the Wider Food Industry. Journal of Agricultural and Food Chemistry. 2005;53:1309-16. [CrossRef] | [Google Scholar]
- Yoo EH, Lee S-Y. Glucose Biosensors:An Overview of Use in Clinical Practice. Sensors. 2010;10(5):4558-76. [CrossRef] | [Google Scholar]
- Yu D, Blankert B, Vire JC, Kauffmann JM. Biosensors in Drug Discovery and Drug Analysis. Analytical Letters. 2005;38:1687-701. [CrossRef] | [Google Scholar]
- Griesser HJ. Thin Film Coatings for Biomaterials and Biomedical Applications. Woodhead Publishing series in Biomaterials. 2016:110 [CrossRef] | [Google Scholar]
- Skinner J, Kay P. Commentary:Metal on metal hips. BMJ. 2011;342 [CrossRef] | [Google Scholar]
- Mei X, Ye D, Zhang F, Di C J. Implantable application of polymer-based biosensors. Journal of Polymer Science. 2021;60(3):328-47. [CrossRef] | [Google Scholar]
- Fang X, Bando Y, Gautam UK, Ye C, Golberg DV. Inorganic semiconductor nanostructures and their field-emission applications. Journal of Materials Chemistry. 2008;18:509522 [CrossRef] | [Google Scholar]
- Han XD, Zhang YF, Zheng K, Zhang XN, Zhang Z, Hao YJ, Guo XY, Yuan J, Wang ZL, et al. Low-Temperature in situ Large strain Plasticity of Ceramic SiC Nanowires and its atomic-Scale Mechanism. Nano Letters. 2007;7(2):452-7. [PubMed] | [CrossRef] | [Google Scholar]
- Su L, Wang H, Niu M, Fan X, Ma M, Shi Z, et al. Ultralight, Recoverable, and High-Temperature-Resistant SiC Nanowire Aerogel. ACS Nano. 2018;2(4):3103-11. [CrossRef] | [Google Scholar]
- Wang X, Uchiyam S. Polymers for Biosensors Construction. State of the Art in Biosensors-General AspectsInTech. 2013 [CrossRef] | [Google Scholar]
- Malhotra BD, Ali M A. Chapter 1-Nanomaterials in Biosensors:Fundamentals and Applications. Namomaterials for Biosensors Fundamentals and Applications Micro and Nano Technologies. 2018:1-74. [CrossRef] | [Google Scholar]
- Bange A, Halsall HB, Heineman WR. Microfluidic immunosensor systems. Biosensors and Bioelectronics. 2005;20(12):2488-503. [CrossRef] | [Google Scholar]
- Carrara S. Nano-Bio-Technology and Sensing Chips:New Systems for Detection in Personalized Therapies and Cell Biology. Sensors. 2010;10(1):526-43. [CrossRef] | [Google Scholar]
- Homola J. Surface Plasmon Resonance Sensors for Detection of Chemical and Biological Species. Chem Reviews. 2008;108(2):462-93. [CrossRef] | [Google Scholar]
- Bard AJ, Faulkner LR. Electrochemical methods:Fundamentals and Applications. John Wiley and Sons. 2000 ISBN:978-0-471-04372-0 [CrossRef] | [Google Scholar]
- Weissleder R. Molecular Imaging in Cancer. Science. 2006;312(5777):1168-71. [CrossRef] | [Google Scholar]
- Oliveros A, Guiseppi-Elie A, Saddow SE. Silicon carbide:a versatile material for biosensor applications. Biomedical Microdevices. 2013;15:353-68. [CrossRef] | [Google Scholar]
- Saddow SE. Recent Advances in SiC biomedical devices:Healthcare Applications, Elsevier:Amsterdam. 2022:1-48. ISBN 9780323906098 [CrossRef] | [Google Scholar]
- Saddow SE. Silicon carbide technology for Advanced Human Healthcare Applications. Micromachines. 2022;13:346 [CrossRef] | [Google Scholar]
- Saddow SE. Silicon Carbide Technology for Advanced Human Healthcare Applications. Elsevier:Amsterdam. 2022 [CrossRef] | [Google Scholar]
- Coletti C, Jaroszeski M, Pallaoro A, Hoff A, Iannotta S, Saddow SE, et al. Biocompatibility and wettability of crystalline SiC and Si surfaces. Proceedings of the 29 Annual International Conference of the IEEE Engineering in Medicine and Biology Society. 2007:5849-52. [CrossRef] | [Google Scholar]
- Saddow SE. Silicon Carbide Biotechnology:A biocompatible semiconductor for Advanced Biomedical devices and Applications (1 ed.). Elsevier:Amsterdam, The Netherlands. 2012 [CrossRef] | [Google Scholar]
- Saddow SE. Silicon Carbide Biotechnology:A biocompatible semiconductor for Advanced Biomedical devices and Applications, (2nd ed.). Elsevier:Amsterdam, The Netherlands. 2016 [CrossRef] | [Google Scholar]
- Bluet JM, Botsoa J, Zakharko Y, Geloen A, Alekseev S, Marty O, et al. Chapter 11-SiC as a Biocompatible Marker for Cell Labelling. Silicon Carbide Biotechnology. 2012:377-429. [CrossRef] | [Google Scholar]
- Chaniotakis N, Sofikiti N. Novel semiconductor materials for the development of chemical sensors and biosensors:A review. Analytica Chimica Acta. 2008;615(1):1-9. [CrossRef] | [Google Scholar]
- Chauhan N, Maekawa T, Kumar D. Graphene based biosensors—Accelerating medical diagnostics to new-dimensions. Journal of Materials Research. 2017;32(15):2860-82. [CrossRef] | [Google Scholar]
- Chen F, Qing Q, Xia J, Tao N. Graphene Field-Effect Transistors:Electrochemical Gating, Interfacial Capacitance, and Biosensing Applications. Chemistry-An Asian Journal. 2010;5:2144-53. [CrossRef] | [Google Scholar]
- . The neuron-semiconductor interface, in Bioelectronics:from theory to applications. Weinheim. 2005:339-93. [CrossRef] | [Google Scholar]
- Godignon P. SiC Materials and Technologies for Sensors Development. Material Science Forum. Array;5:Array-14. [CrossRef] | [Google Scholar]
- Holzinger M, Goff A, Cosnier S. Nanomaterials for biosensing applications:a review. Frontiers in Chemistry. 2014;63(1-10) [CrossRef] | [Google Scholar]
- Lebedev AA, Davydov SY, Eliseyev IA, Roenkov AD, Avdeev O, Lebedev SP, et al. Graphene on SiC Substrate as Biosensor:Theoretical Background, Preparation, and Characterization. Materials. 2021;14(3):590 [CrossRef] | [Google Scholar]
- Mahmoodi M, Ghazanfari L. Silicon Carbide:A Biocompatible Semiconductor Used in Advanced Biosensors and BioMEMS/NEMS. Physics and Technology of Silicon Carbide Devices InTech. 2012 [CrossRef] | [Google Scholar]
- Mohanty SP, Kougianos E. Biosensors:a tutorial review. IEEE Potentials. 2006;25(2):35-40. [CrossRef] | [Google Scholar]
- Pohanka M. The Piezoelectric Biosensors:Principles and Applications, a Review. International Journal of Electrochemical Science. 2017;12:496-506. [CrossRef] | [Google Scholar]
- Paul J, Lim WM, O’Cass A, Hao AW, Bresciani S. Scientific procedures and rationales for systematic literature reviews (SPAR-4-SLR). International Journal of Consumer Studies. 2021;45(4):1-16. [CrossRef] | [Google Scholar]
- Comerio N, Strozzi F. Tourism and its economic impact:A literature review using bibliometric tools. Tourism Economics. 2019;25(1):109-31. [CrossRef] | [Google Scholar]
- Norris M, Oppenheim C. Comparing alternatives to the web of Science for coverage of the social sciences literature. Journal of Informetrics. 2007;1(2):161-9. [CrossRef] | [Google Scholar]
- Van Eck NJ, Waltman L. Citation-based clustering of publications using CitNetExplorer and VOSviewer. Scientometrics. 2017;111(2):1053-70. [CrossRef] | [Google Scholar]
- Callon M, Courtial JP, Turner WA, Bauin S. From translations to problematic networks:An introduction to co-word analysis. Social Science Information. 1983;22(2):191-235. [CrossRef] | [Google Scholar]
- Castriotta M, Loi M, Marku E, Naitana L. What’s in a name? Exploring the conceptual structure of emerging organizations. Scientometrics. 2019;18(2):407-37. [CrossRef] | [Google Scholar]
- Donthu N, Kumar S, Mukherjee D, Pandey N, Lim WM. How to conduct a bibliometric analysis:An overview and guidelines. Journal of Business Research. 2021;133:285-96. [CrossRef] | [Google Scholar]
- Newman MEJ, Girvan M. Finding and Evaluating Community Structure in networks. Physical Review E. 2004;69(2):026113 [CrossRef] | [Google Scholar]
- Pesta B, Fuerst J, Kirkegaard E. Bibliometric Keyword Analysis across Seventeen Years (2000–2016) of Intelligence Articles. Journal of Intelligence. 2018;6(4):46 [CrossRef] | [Google Scholar]
- Uusalo E, Yli-Urpo A. From contacts and bondings between bone and bioactive glass to bonding of bioactive glass and porcelain to metal alloys, different methods of fracture repair. Proceedings of the Finnish Dental Society. 1991;87(2):299-308. [PubMed] | [Google Scholar]
- Anzalone R, Severino A, Locke C, Rodilosso D, Cristina T, Saddow SE, et al. 3C-SiC Hetero-Epitaxial Films for Sensors Fabrication. Advances in Science and Technology 2008. [CrossRef] | [Google Scholar]
- Nakao S, Ando T, Chen L, Mehregany M, Sato K. Mechanical characterization of SiC film at high temperatures by tensile test. IEEE 21st International Conference on Micro Electro Mechanical Systems. 2008:447-50. Available from:https://ieeexplore.ieee.org/abstract/document/4443689
[CrossRef] | [Google Scholar] - Sangsu N, Seo JH, Lee E. The Fabrication by using Surface MEMS of 3C-SiC Micro-heaters and RTD Sensors and their Resultant Properties. Transactions on Electrical and Electrical Materials. 2009;10(4):131-4. [CrossRef] | [Google Scholar]
- Heon-Jin C, Han-Kyu S, Jung-Chul L, Yun-Mo S. Growth and modulation of Silicon Carbide nanowires. Journal of Crystal growth. 2004;269(2-4):472-8. [CrossRef] | [Google Scholar]
- Peng Y, Xili T, Guizhen W, Zhe G, Xiangyun G, Yong Q, et al. NiO/SiC Nanocomposite Prepared by Atomic Layer Deposition Used as a Novel Electrocatalyst for Nonenzymatic Glucose Sensing. ACS Applied Materials and Interfaces. 2015;7(8):4772-4. [CrossRef] | [Google Scholar]
- Yakimova R, Steinhoff G, Petoral RM, Vahlberg C, Khranovskyy V, Yazdi GR, Uvdal K, Spetz A, et al. Novel material concepts of transducers for chemical and biosensors. Biosensors and Bioelectronics. 2007;22(12):2780-5. [CrossRef] | [Google Scholar]
- Sebastian JS, Matthias S, Alexandra O, John H, Martin S, Martin SB, Christopher LF, Stephen ES, Ian DS, et al. Organic Functionalization of 3C-SiC Surfaces. ACS Applied Materials Interfaces. 2013;5(4):1393-9. [CrossRef] | [Google Scholar]
- Yao G, Zhang L, Song Q, Zhang R, Zhao F, Li W, et al. Simultaneously enhancing mechanical and tribiological properties of carbon fiber composites by grafting SiC hexagonal nanopyramids for brake disk application. Journal of Materials Science and Technology. 2022;121:1-8. [CrossRef] | [Google Scholar]
- Zhang M, Jiao D, Tan G, Zhang J, Wang S, Wang J, et al. Strong, Fracture-Resistant Biomimetic Silicon carbide composites with Laminated Interwoven Nanoarchitectures Inspired by the Crustacean Exoskeleton. ACS Applied Nanomaterials. 2019;2:1111-1119. [CrossRef] | [Google Scholar]
- Zhou YS, Ikeuchi K, Ohashi M. Comparison of the friction properties of four ceramic materials for joint replacement. Wear. 1997;210(1-2):171-7. [CrossRef] | [Google Scholar]
- Srivastava ON, Srivastava A, Dash D. Synthesis, Charaterization and applications of some nanomaterials (TiO2 and SiC nanostructured films, organized CN structures, ZnO structures and CNT-blood platelet clusters). Pramana. 2005;65:581-92. [CrossRef] | [Google Scholar]
- Leung I, Guo W, Friedman I, Gleason J. Natural occurrence of silicon carbide in a diamondiferous kimberlite from Fuxian. Nature. 1990;346:352-4. [CrossRef] | [Google Scholar]
- Bolz A, Schaldach M. Physikalische Mechanismen der Festkörper-Protein-Wechselwirkung an der Phasengrenze a-SiC:H-Fibrinogen-Physical Mechanisms of Solid-Protein Interactions at the Interface between Amorphous Silicon Carbide and Fibrinogen. Biomedical Engineering /Biomedizinische Technik. 1992;37(11):244-53. [CrossRef] | [Google Scholar]
- Monnink SH, van Boven AJ, Peels HO, Tigchelaar I, de Kam PJ, Crijns HJ, van Oeveren W, et al. Silicon-carbide coated coronary stents have low platelet and leukocyte adhesion during platelet activation. Journal of Investigative Medicine. 1999;47(6):304-10. [PubMed] | [Google Scholar]
- Kalnins U, Erglis A, Dinne I, Kumsars I, Jegere S. Clinical outcomes of silicon carbide coated stents in patient with coronary disease. International Medical Journal of Experimental and Clinical Research. 2002;8(2):PI16-20. [PubMed] | [Google Scholar]
- Sun X, Li C, Wong W, Wong N, Lee C, Lee S, Teo B, et al. Formation of Silicon Carbide Nanotubes and Nanowires via Reaction of Silicon (from Disproportionation of Silicon Monoxide) with Carbon Nanotubes. Journal of the American Chemical Society. 2002;124(48):14464-71. [CrossRef] | [Google Scholar]
- Shim HW, Huang H. Nanowebs and Nanocables of silicon carbide. Nanotechnology. 2007;18(33):335607 [CrossRef] | [Google Scholar]
- . Chapter 4-Graphene Functionalization for Biosensor Applications. Silicon Carbide Biotechnology. 2016 [CrossRef] | [Google Scholar]
- Acedo FJ, Barroso C, Casanueva C, Galan JL. Co-Authorship in Management and Organizational Studies:An Empirical and Network Analysis. Journal of Management Studies. 2006;43(1):957-83. [CrossRef] | [Google Scholar]
- Cisneros L, Ibanescu M, Keen C, Lobato-Calleros O. Array. 2018;117(2):919-951. Akadémiai Kiadó [CrossRef] | [Google Scholar]
- Williams EH. Immobilization of streptavidin on 4H–SiC for biosensor development. Applied Surface Science. 2012;258(16):6056-63. [CrossRef] | [Google Scholar]
- Menaa F, Fatemeh Y, Vashist SK, Iqbal H, Sharts ON, Menaa B, et al. Graphene, an Interesting Nanocarbon Allotrope for Biosensing Applications:Advances, Insights, and Prospects. Biomedical Engineering and Computational Biology. 2021:12 [CrossRef] | [Google Scholar]
- Zorman CA, Parro R. Micro-and nanomechanical structures for silicon carbide MEMS and NEMS. Physica status solidi (b). 2008;245(7):1404-24. [CrossRef] | [Google Scholar]
- Xu Y, Hu X, Kundu S, Nag A, Afsarimanesh N, Sapra S, et al. Silicon-Based Sensors for Biomedical Applications:A Review. Sensors. 2019;19(13):2908 [CrossRef] | [Google Scholar]
- Li PCH. Microfluidic Lab-on-a-chip for Chemical and Biological Analysis and Discovery. Chromatographic Science series. 2005;94 [CrossRef] | [Google Scholar]
- Huang X, Zhu Y, Kianfar E. Nano Biosensors:Properties, applications and electrochemical techniques. Journal of Materials Research and Technology. 2021;12:1649-72. [CrossRef] | [Google Scholar]